This article is a companion piece to my previous articles titled, A Paradigm Shift in Bioenergetics and Applied Bioenergetics.
In discussions about energy systems, coaches often make rigid distinctions between the different modes of energy production, discussing anaerobic and aerobic metabolism as if they are entirely compartmentalized processes that occur in isolation. Through this worldview they see things as black or white — aerobic or anaerobic. In figure I, you’ll find two examples depicting this view of energy systems.
I suspect that many of you are familiar with these images, or images like these. You’ll often find them in coaching manuals, undergraduate exercise science textbooks, and personal training certification courses. In essence, these images are depicting the percentage of energy contribution from the various energy pathways on the Y-axis with a time scale on the x-axis. According to these charts, a sustained burst of power lasting under two seconds is largely powered by ATP stores, between two and ten seconds we are relying on phosphagen stores to keep us going, from ten seconds to two minutes the glycolytic system takes over, and then anything over two minutes is ‘aerobic’.
Off the bat we can already poke holes in this model. For example, these proponents of this system assert that phosphocreatine supplies almost all of the energy needed for sustained bursts of contraction lasting less than ten seconds, after which it is replaced by glycogenolysis. This seems well and good, but it’s in direct conflict with contemporary biochemical literature. For example, in Robert Shulman and Douglas Rothman’s paper titled, The “glycogen shunt” in exercising muscle: A role for glycogen in muscle energetics and fatigue, they report the presence of the enzyme glycogen phosphorylase (GP) in its active form during exercise under conditions where glycogen concentrations are constant. This seems illogical at first glance given that the role of GP is the key enzyme for breaking down muscle and liver glycogen stores to release glucose. The only way for GP to be found in it’s active form while glycogen concentrations are stable is if glycogen synthesis and breakdown are occurring in tandem with one another. This would only make sense if sustained muscle contractions require continual phosphocreatine breakdown — in this case GP would need to rapidly increase activity to restore phosphocreatine and ATP. Through the classic lens of bioenergetics this would seem paradoxical since it is believed that phosphocreatine consumption drops after ten seconds. However, in Yourgran Chung and colleagues' paper titled, Metabolic Fluctuation During A Muscle Contraction Cycle, we see that phosphocreatine consumption is approximately forty times greater than previously believed. This is the first nail in the coffin for the classic model of energy systems referenced in figure I.
The second nail comes from Kevin McNully and colleagues’ paper, Simultaneous In Vivo Measurements Of HBO2 Saturation and PCr Kinetics After Exercise In Normal Humans.
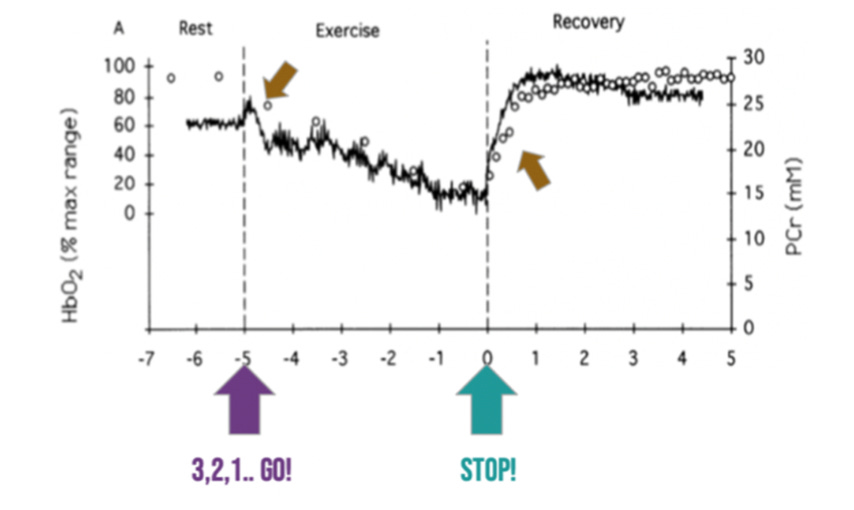
In McNully et al’s paper we see that phosphocreatine and oxygen kinetics are tightly coupled during exercise and following exercise. There are also reports by Paul Greenhaff and James Timmons where they state, “However, that PCr hydrolysis and lactate production do not occur in isolation, and that both are initiated rapidly at the onset of contraction.” Whereas the classic model of bioenergetics is compartmentalized, we now need to consider the fact that all energetic processes overlap in the millisecond time scale. In essence, we can rightfully say that all training is aerobic and lactic, which is a sentiment I expressed in great depth in a past article titled, A Paradigm Shift in Bioenergetics: A New Way Of Thinking About Energy Systems.
This often seems counter intuitive to people, especially those that come from a track & field background where we are often told that sprinters are anaerobic athletes and the long distance runners are aerobic athletes. In a sense, the way that energy systems are often explained is all backward.
In figure III you’ll find a NIRS measurement recorded from an athlete performing a 60-second sprint on an air bike. Notice that the second they begin their sprint, oxygen is utilized instantaneously in the working muscle. In fact, oxygen is consumed at a much greater rate than it is supplied to the active muscle, which is why muscle oxygen saturation is declining. As soon as the athlete stops pedaling oxygen supply supersedes oxygen utilization, and oxygen saturation rises rapidly. Among other things, this tells us that Oxygen is utilized immediately upon loading. That is to say, that the energy contribution from oxidative processes are quite high at the start of a sprint, and they decrease over the course of the sprint as muscle oxygen supplies are depleted. This is the opposite of what you would see in the classic charts. Furthermore, when oxygen is depleted we can infer that less fat is being oxidized for fuel which is discussed in depth in a past article, and that glycolysis is increasingly being relied upon to power activities (demonstrated in figure IV).
However, because glycolysis is an extremely inefficient fuel source most athletes are quick to reach volitional failure when muscle oxygen supplies are depleted. When this occurs we’ll often see elevated blood lactate concentrations. The reason for this is that lactate the more sugars are broken down, the higher blood lactate levels will rise. Additionally, lactate also buffers the acidic hydrogen ions that are produced during the formation of glucose. Despite the fact that lactate accumulation occurs at the point of failure, it is not a cause of failure. Figure V does a great job of showing the relationship between muscle pH (increased hydrogen ions = lower pH) and lactate.
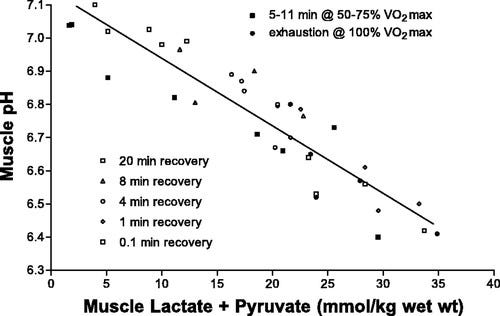
Where this leaves us is that sprinting is not only aerobic, but it is also lactic. When we begin sprinting muscle oxygen saturation levels immediately drop as oxygen is utilized at a much faster rate than it is supplied to the working muscles (mind you, phosphocreatine is being consumed at a fast rate as well). Once muscle oxygen saturation stores bottom out, we begin to increasingly rely on glycolytic processes to keep us going. When this occurs we also see elevated in blood lactate. However, because glycolysis is an extremely inefficient mode of energy production, we cannot operate for long with low oxygen supplies. In effect, the classic view of sprint bioenergetics is all backwards !
If you enjoyed reading this article please consider subscribing to the Emergent Performance Lab Substack.
Free subscribers can expect content short form posts sporadically throughout the month, as well as previews of my long form paid content.
Paid subscribers will receive 1-2 content pieces per week including one long form exclusive content piece every Sunday, as well as bonus content including mini-articles, case studies, monthly Q&A’s, and podcast.